Explore our innovative services designed to shape the future through the lens of our past. Discover how our project management solutions can revolutionize your business.
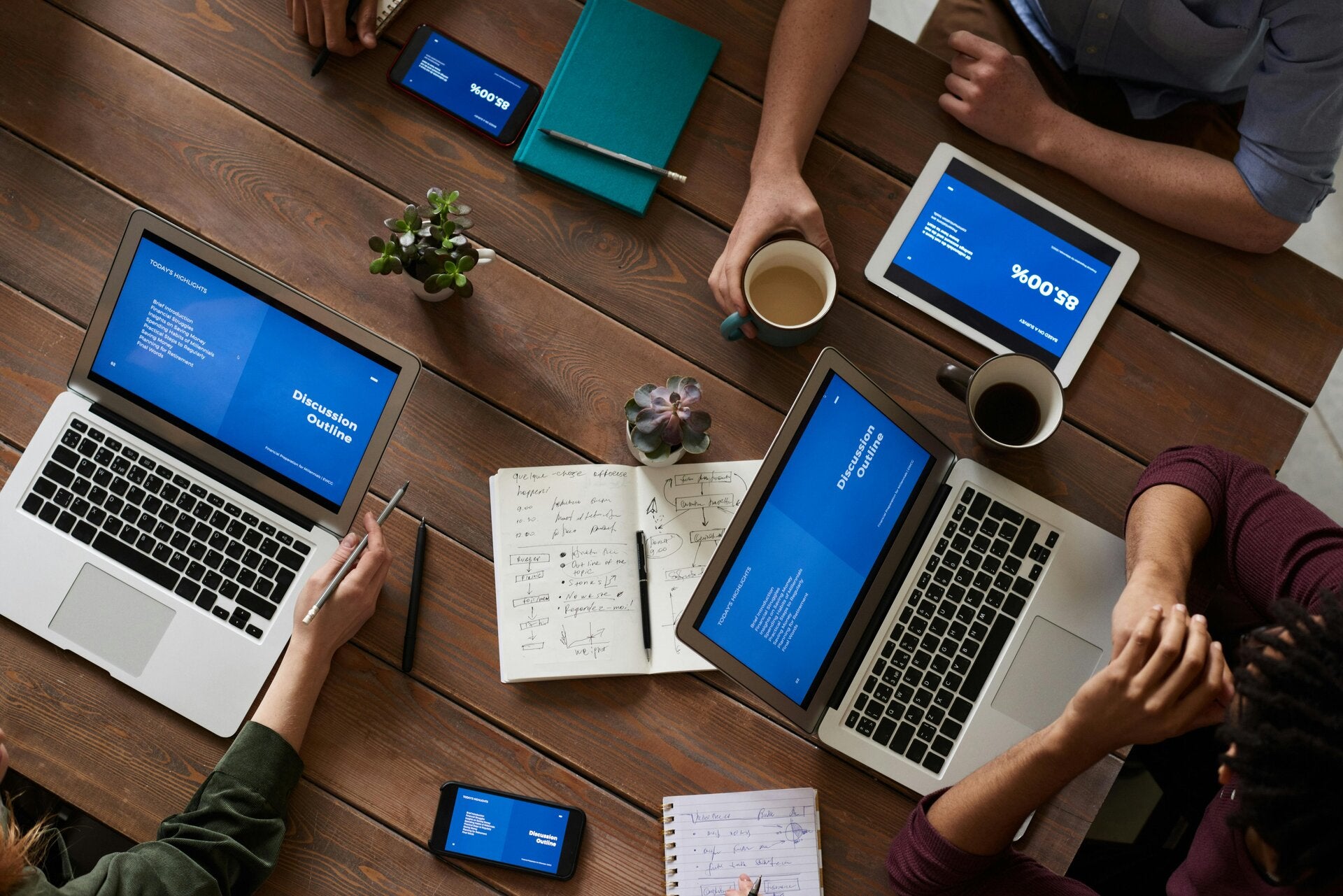
University Driven research and development.
Core Pillars of Pharaohverse
1. Reclamation of History
What they told us were tombs… were test beds.
• We reclaim ancient wonders from centuries of misinterpretation.
• We view pyramids, sarcophagi, and rituals as part of a highly advanced human endeavor, not primitive superstition.
• The Saqqara Bird, the precision-cut stone, and the fire rituals — all evidence of aerospace curiosity and sacred science.
⸻
2. Human Flight is Sacred
They didn’t dream of flying. They did it.
• The Pharaohs were the first true pilots — not just metaphorically.
• Sarcophagi as G-suits. Pyramids as launch complexes. Ancient rituals as literal instructions.
• Flight is freedom, ascension, and balance — and it’s a birthright.
⸻
3. Myth Meets Mechanism
We combine sacred symbolism with functional design.
• Our tech is informed by ancient forms, natural forces, and aerodynamic truths.
• From EM launch systems, to solar glidecraft, to AI-optimized birds of flight, Pharaohverse is the fusion of the mystical and mechanical.
⸻
4. Energy is Limitless, Movement is Free
We don’t run on gas. We run on light, wind, and will.
• The future doesn’t require fossil fuels. It requires ancient insight and modern application.
• Solar-powered, gliding, AI-stabilized personal flight systems that let you:
• Hop across cities.
• Cross countries.
• Float like a hawk, move like a jet, or drift like the gods.
⸻
5. Build the Future By Living the Lore
The Pyramid wasn’t the end. It was the prototype.
• The pharaohs left behind a roadmap. It’s our job to decode it, live it, and build forward.
• Pharaohverse is a movement: artistic, technological, mythological, and aspirational.
• We don’t just sell shirts or build aircraft. We awaken a civilization-wide dream.
This proposal outlines a bold interdisciplinary research project to re-examine Old Kingdom pyramids and funerary practices through the lens of aerospace engineering and high-energy physics. We hypothesize that key elements of ancient Egyptian royal burial – including pyramid structures, rituals, and burial equipment – may have served practical technological functions analogous to a launch system designed to propel the pharaoh’s spirit (or even body) into the sky. In other words, we propose that pyramids were not merely symbolic tombs but were operational prototypes for high-energy transformation or “launch” devices. This visionary hypothesis challenges conventional Egyptology, yet it is grounded in intriguing evidence:
• Aerodynamic model artifact: The Saqqara Bird, a wooden figure long thought a toy or ritual object, exhibits aerodynamic properties suggestive of flight experimentation . Wind-tunnel tests show it generates lift and, with a small tailplane added, it can glide stably . This hints that ancient Egyptians may have had practical knowledge of aerodynamics.
• Ritual interpreted as survival protocol: The “Opening of the Mouth” ceremony – traditionally to reanimate the deceased’s senses – can be reinterpreted as ensuring the ability to breathe during extreme conditions (as modern astronauts must) . It may reflect a form of breath control or pressure equalization ritual needed for a launch.
• Protective burial equipment as G-force gear: The gold sarcophagus and tight linen mummy wrappings that encased pharaohs strongly resemble the protective capsule and pressure suits used in aerospace today. Linen bandages were resin-soaked and wrapped so tightly that they “kept everything together” and prevented the body from distorting or disintegrating – much like a modern G-suit stabilizes an astronaut’s body under high acceleration. The gold coffin, aside from its ritual symbolism, is a superb thermal and radiation shield (gold is used in spacecraft for heat protection ) around the pharaoh’s body .
• Architectural alignments and materials: The Great Pyramid’s internal passages are angled at ~26.5° , a shallow incline that intriguingly matches calculated launch trajectories more than vertical ascent. Narrow shafts (“air shafts”) aim toward the sky and were sealed off – possibly to channel forces or exhaust. The extensive use of Aswan granite (8,000+ tons) in the King’s Chamber and its chambers is notable: granite is far more heat-resistant and structurally robust than limestone, hinting that these chambers were engineered to withstand intense energy, pressure, or heat. Additionally, traces of burnable resins and oils used in burial rites (hundreds of liters in some tombs) suggest a potential fuel source. Some researchers have even posited that fires were lit in sealed pyramid shafts during construction – possibly a test or component of an energetic reaction.
• Textual evidence of literal ascent: The Pyramid Texts, inscribed in 5th–6th Dynasty pyramids, repeatedly describe the pharaoh flying or ascending to the sky in strikingly literal terms. For example, “May you ascend to the sky, may the sky give birth to you like Orion…” and “O king, you are this Great Star, the companion of Orion, who traverses the sky…” . While traditionally understood metaphorically, such lines could be read as eyewitness-like accounts of a launch or “ascent to the heavens.”
• Iterative prototyping in pyramid design: The evolution from Djoser’s Step Pyramid through Sneferu’s “failed” projects (Meidum’s collapsed pyramid and the Bent Pyramid at Dahshur) to the perfected Great Pyramid of Khufu can be viewed as a sequence of engineering trials. The Bent Pyramid’s sudden change of angle (from 54° to 43° half-way up) was likely a structural adjustment to prevent collapse – analogous to a rocket design being modified after early tests. Sneferu’s next pyramid (the Red Pyramid) was built fully at the safer 43° slope , confirming a learning process. These “failures” and refinements are consistent with an R&D trajectory toward a stable, functional launch platform. The Great Pyramid, with its precise alignment and added features (Grand Gallery, angled shafts, massive granite components), may represent the culmination of this ancient engineering project.
Conclusion of Summary: We respectfully propose a thorough investigation of this hypothesis, bringing together Egyptologists with aerospace engineers, physicists, materials scientists, and historians of technology. The goal is not to diminish the spiritual achievements of the pyramid builders, but to enrich our understanding by exploring whether advanced practical knowledge underpinned their religious expressions. The following sections detail the evidence, research plan, and methodologies for testing this hypothesis in a rigorous, scientific manner – all while honoring the cultural context and expertise of Egyptology.
⸻
Background and Rationale
Ancient Egyptian civilization is renowned for its visionary achievements in architecture and ritual. Traditionally, Egyptologists interpret the pyramids as tombs designed to protect the pharaoh’s body and symbolize his ascension to the afterlife. Funerary ceremonies, like the Opening of the Mouth, are seen as purely religious practices to restore the deceased’s faculties for the afterlife . However, history has shown that pre-modern cultures sometimes encoded surprisingly practical or scientific knowledge in symbolic form. We ask: Could the Egyptians have intentionally engineered their pyramids and rites with dual purposes – spiritual and technological?
Several factors prompt this question. First, the physical scale and precision of pyramid construction exceed apparent functional needs if they were only tombs. The Great Pyramid’s builders demonstrated masterful control of materials and angles: its descending and ascending corridors form a consistent ~26.5° incline , and its massive granite beams in the King’s Chamber suggest an intent to contain great force or weight . Such choices go beyond what is necessary for a symbolic monument, hinting at an underlying functional design.
Second, certain anomalous artifacts and texts beg for a fresh perspective. The Saqqara Bird (a small wooden model discovered in 1898) is one example. With a wingspan of 18 cm and a shape uncannily like a glider, it has puzzled researchers: mainstream views call it a toy or ritual object, yet it lacks painted feathers and has an aerodynamic form . In 2006, aerodynamicist Simon Sanderson tested a replica of the Saqqara Bird in a wind tunnel and found it produced four times its own weight in lift – a remarkable result. When a stabilizing tail (hypothesized to have been lost from the original) was added, the model flew stably in a flight simulator under conditions akin to the Nile valley winds . Modern technology thus demonstrated that 2,200 years ago an Egyptian craftsman carved a shape that could glide. This raises the possibility that knowledge of aerodynamics existed in ancient Egypt, but was expressed in artistic or ritual objects due to the lack of a known practical context for flight.
If Egyptian artisans and priests held secret technical knowledge, what might have been their grandest experiment? We propose it was the pyramid complex itself – a holistic system to “launch” the divine king into the heavens. Launch, in this context, could mean a physical propulsion or a controlled energetic release envisioned as aiding the pharaoh’s soul to literally reach the stars (the Pyramid Texts often identify the king with stars and make reference to a “stairway to heaven” ). The Great Pyramid of Khufu, in particular, contains features (angled passages, “star shafts,” hermetically sealed chambers, conductive materials like gold and copper fittings) that align with what one might design if the goal were to generate and direct force or energy upward.
Critically, this hypothesis does not require attributing supernatural or anachronistic capabilities to the ancient Egyptians. Instead, it suggests they may have been experimenting within the laws of physics as they understood them, using the materials at hand – much as other ancient cultures experimented with pneumatics, steam power (e.g. Hero of Alexandria), or primitive batteries (the Baghdad battery). In Egypt’s case, the stakes were higher: the pharaoh’s resurrection and eternal life were on the line, providing a powerful impetus to attempt the extraordinary.
By re-reading the archaeological evidence with “technological eyes,” we might explain longstanding mysteries: Why do some pyramids show signs of internal scorching or chemical residues? Why devote enormous resources to mining and transporting thousands of tons of granite for chambers deep inside a pyramid ? Why include narrow shafts that lead to nothing visible outside, angled precisely at certain celestial targets? Our hypothesis offers a unifying answer: these were components of a launch mechanism – part spiritual, part physical – intended to transform the king and send him to the stars.
Evidence Supporting the Hypothesis
1. The Saqqara Bird and Ancient Aerodynamics
Front view of the wooden “Saqqara Bird” artifact (Late Period, ca. 200 BCE). Researchers discovered that this object, when tested as a scale-model glider, can produce lift and glide, suggesting an ancient Egyptian exploration of aerodynamic principles .
One cornerstone of our hypothesis is that the ancient Egyptians may have dabbled in aerodynamics and prototypical flight. The Saqqara Bird artifact provides compelling evidence of this possibility. Discovered in the tomb of Pa-di-Imen at Saqqara in 1898 and dated to ~200 BCE, it is a carved sycamore wood figure of a bird with outstretched, aerodynamically contoured wings (span ~18 cm). Notably, it has no legs and an upright tail, unlike a purely decorative bird carving . This shape led Dr. Khalil Messiha in the 1970s to speculate it was a model glider rather than a toy .
Modern tests have borne out the aerodynamic capability of the Saqqara Bird. As mentioned, a wind-tunnel test by Sanderson found the model generates substantial lift even without a tail stabilizer . With the addition of a small tailplane (as Messiha theorized was originally present), the replica was shown in a controlled simulation to fly properly, maintaining stable glide in conditions similar to the Egyptian sky . According to a History Channel analysis, “over 2,000 years after the ancient Egyptians carved this mysterious bird, modern technology has proved beyond doubt that it could have flown.” . While this artifact comes from a period later than the pyramids, it demonstrates an interest in and knowledge of flight within Egyptian culture.
We propose to leverage this clue by treating the Saqqara Bird as a conceptual predecessor to the launch system. In a ritual context, it might have been a symbolic “ba” bird or solar falcon. But functionally, it could represent experimentation with sending something aloft – a small step from which the idea of sending a king’s soul to the sky via mechanical means might grow. The presence of such a glider model suggests that the idea of human flight was not unthinkable in ancient Egypt. We will further investigate the Saqqara Bird by building high-fidelity replicas (employing 3D scans and lightweight materials) and flying them with varying tail designs to conclusively document its aerodynamic performance. This will be done in collaboration with aerospace engineers and will be fully documented.
2. Reinterpreting Funerary Rituals as Operational Protocols
Ancient Egyptian mortuary rituals were rich in symbolism. Our hypothesis suggests some rituals may also have had practical preparations embedded within them, analogous to pre-launch checklists or physiologic conditioning for astronauts.
The Opening of the Mouth ritual is particularly thought-provoking. In this ceremony, priests ceremonially touched the mummy’s mouth, eyes, and other senses with special instruments (such as a ritual adze and the “peseshkef” blade) to restore the ability to breathe, see, and speak in the afterlife . Texts explicitly state its purpose was to give the deceased their fundamental senses back so they could function in the next world – “Vital functions such as breathing, speaking, seeing, eating, and drinking were among these necessities.” .
From a symbolic standpoint, this ensured the pharaoh could breathe and communicate upon reaching the afterlife. From an engineering standpoint, however, this ritual can be seen as addressing the very real concern of sustaining life (or at least integrity) under traumatic conditions. High acceleration and rapid ascents pose serious challenges to breathing – modern pilots and astronauts train extensively in breathing techniques (like the anti-G straining maneuver) to avoid blackout. If the pyramid were to serve as a launch device generating sudden forces or even rapid ascension of the soul, ensuring an “open airway” and the capacity for breath would be crucial. The ritual might be the esoteric reflection of a practical measure: perhaps the priests or embalmers repositioned the pharaoh’s head and neck to keep the airway open, or included medical knowledge of resuscitation.
Additionally, the Opening of the Mouth ritual involved burning incense to purify the air and appease the gods . Incense (often resin-based) produces smoke – which could also serve a mundane purpose as a visual indicator or medium (for example, filling a chamber with smoke to observe airflow or leakage, as modern engineers do in pressure testing). While this is speculative, it is conceivable that ritual incense burning doubled as a test of the pyramid chamber’s sealing (did smoke escape?) or as a way to create a certain atmospheric condition inside before “ignition.”
In essence, we will re-examine funerary ritual scenes and residue on mummies for any signs of practical actions. Was the pharaoh’s throat physically propped open with some device? Were aromatic resins chosen for their chemical properties (e.g., releasing oxygen when heated)? Did the texts preserve any “instructions” that could be read as operating procedures? We will consult both Egyptologists and medical experts in ancient medicine on this point.
3. Mummy Wrappings and Gold Sarcophagi as G-Suits and Capsules
The mummification process and burial equipment of the pharaoh can be likened to the safety equipment of a cosmonaut – if we view it through a technological lens.
Linen bandages and resin: The body of the king was wrapped in hundreds of meters of linen, often in dozens of layers, to the point of rigidity. As described in modern analyses, “the embalmers began wrapping the body as a whole… As they applied new layers, the embalmers coated the linen with hot resin to glue the bandages in place.” . The result was a cocoon that “kept everything together. Without this binding system, the fragile, desiccated mummies would likely burst or fall apart.” . This statement is remarkably analogous to the purpose of a flight G-suit: to keep the body’s tissues in place and prevent internal damage under stress. The tight wrapping and resin impregnation would have stabilized the pharaoh’s body, preventing sudden decompression or disintegration if subjected to force. In our hypothesis, the mummy bandages and resin form a primitive pressure suit, maintaining the pharaoh’s form during the violent process of launch or energy release. It is also worth noting that the bandages were resin-soaked – resins and bitumen are chemically energy-rich. If one were designing a system to immolate or provide thrust, encasing the “fuel” with the payload (the body) is conceivable, albeit gruesome. We do not claim the Egyptians set their kings on literal fire as fuel; rather, the resin-soaked wrappings might have had a dual role of preservation and planned combustion or sublimation in a high-energy event. We will analyze residue on royal mummies for any microscopic evidence of heating or combustion (charring of bandages, soot in resin layers, etc.) that could support this idea, taking care to differentiate ritual anointing from unintended burning.
Gold inner coffin: Pharaohs of the New Kingdom (and likely influential predecessors) were enclosed in anthropoid coffins of gold. Tutankhamun’s famous inner coffin, for example, is 110.4 kg of solid goldforming a tight-fitting shell around the mummy . Gold is an inert metal, excellent for shielding – it does not corrode, it blocks moisture and microorganisms, and importantly, it reflects heat and radiation extremely well (NASA uses thin gold film on astronauts’ visors and satellite instruments to reflect harmful radiation ). In our hypothesis, the gold coffin served as a protective capsule akin to a space capsule or pressure chamber. If a high-energy reaction occurred in the burial chamber (for instance, a flash of intense heat or a surge of electromagnetic energy), the gold lining around the king would safeguard his body or soul. It might keep internal pressure stable for a moment or reflect away thermal energy.
The tight nesting of multiple coffins (wooden outer coffins, inner gold coffin) is also notable – it mirrors the concept of staging and encapsulation. The outer layers could be sacrificial, absorbing shocks or blasts, leaving the innermost (gold) intact. Indeed, the Bent Pyramid complex included two burial chambers and an unusual double entrance , which might be an architectural analog to multi-layered protection or a staged launch where an initial blast is channeled from one chamber to another.
We will engage materials scientists to model how a body wrapped in linens and resins, inside a gold coffin, would fare under different extreme scenarios (rapid acceleration, intense heat, etc.). The exceptional preservation of these royal mummies suggests minimal disturbance by heat or shock – but perhaps that’s exactly because the ancient design succeeded in protecting them during the “transformation.” Any signs of trauma or sudden pressure on the bodies (e.g., fractured bones that could hint at vibration or acceleration injuries) will be looked for via medical imaging, in partnership with osteoarchaeologists and paleopathologists.
4. Pyramid Architecture: Alignments, Angles, and Materials for Launch
The architectural features of the pyramids themselves constitute a major piece of evidence. We focus on the Great Pyramid of Giza as the archetype (as it is the most thoroughly studied), with comparative reference to Sneferu’s pyramids.
Angled passages and “launch tube”: The Great Pyramid’s internal passages have a slope of approximately 26.5°, very close to 26° 18’ (a ratio of 1:2 rise over run) . This angle is shallow relative to the pyramid’s face (which is ~51.5°) and has long been noted for its astronomical alignment – the Descending Passage pointed toward the polar star Thuban at c.2500 BC. In our context, the 26.5° angle is intriguing as a potential launch trajectory. Modern orbital launches do not go straight up; they quickly shallow their ascent to build horizontal velocity. While 26° is lower than a typical rocket’s gravity turn, it could symbolize the desired path for the king’s soul – not a vertical shot, but an inclined ascent aimed at the northern sky (where eternal circumpolar stars reside). If an energetic “push” were given to the king’s spirit, the Grand Gallery and Ascending Passage could act as a guided channel, aiming it upward at that angle. We note that the Ascending Passage leads to the Grand Gallery, a tall and narrow corridor that might have served as an accelerating ramp or housing for some counterweight mechanism (as some engineers theorize for construction). In our hypothesis, the Grand Gallery could be the chamber where energy was built up – perhaps via combustion or pressure – then funneled up the Ascending Passage when a sealed plug (the granite “blocking stones”) was released . Essentially, the pyramid interior might function like a giant cannon or launch tube, with the King’s Chamber at the base serving as a combustion or energy chamber, and the narrow passages directing the force.
Granite in high-stress areas: As documented, the Great Pyramid used limestone for most of its mass but reserved granite (from Aswan) for critical elements: the King’s Chamber walls and roof, the five-layered relieving chambers above it, and the portcullis blocking stones in the Ascending Passage . This granite was transported ~900 km and blocks weighed up to 70–80 tons each , an immense effort if their purpose was merely symbolic. Granite is extremely strong under compression and has a high melting point (~1200°C, vs. ~825°C for limestone which can calcine and crumble under heat). It is plausible that the builders anticipated extreme heat or pressure in these areas – for example, if a chemical reaction or explosive force was generated in the King’s Chamber, only granite could contain it without cracking. We also note that the interior of the Bent Pyramid at Dahshur has two chambers (one corbelled) and some cedar beams reportedly inside – details that might hint at attempts to reinforce or buffer stress in early designs. Our structural engineering analysis will examine whether the distribution of granite correlates with where a high-energy device would need reinforcement (preliminarily, it does: the “relieving” compartments of granite above the King’s Chamber could disperse a sudden force upward, and the massive coffer (sarcophagus) in the chamber, also granite, could endure pressure where a limestone one might shatter).
Narrow shafts and possible propulsion residues: The Great Pyramid’s so-called air shafts in the King’s Chamber run upward at about 45° (south) and with a bend ~32° (north) , exiting on the pyramid’s faces (or originally to the exterior). The Queen’s Chamber shafts (~39° upward, initially sealed) end in small closed “doors” with copper handles. Traditional views say these shafts were symbolic channels for the soul or for ventilation during building . We propose they might have been exhaust or energy release vents. If a blast or combustion happened in a chamber, these shafts would direct excess pressure outwards (rather than blowing apart the core). It is notable that in 1993, a robotics exploration found reddish-brown residue on the floor of the Queen’s Chamber southern shaft’s final cavity . Preliminary analysis identified it as red ochre (iron oxide) used by workers, but we intend to test if any chemical traces of soot, ash, or combustion byproducts are present. A recent study suggests fires may indeed have been lit in those shafts during construction . We will employ advanced chemical analysis (GC-MS, X-ray fluorescence) on the detritus from the shafts (such as the “Dixon relics” chamber) to check for organic compounds from resins or oils that may have combusted. If we find, for instance, traces of cedar oil or bitumen combustion, it would strongly support the notion of a controlled burn having occurred in a sealed context.
Alignment with launch azimuth: A fascinating point arises from ancient star alignment data: The Great Pyramid’s north-south orientation is almost perfect, and its Descending Passage is oriented northward at that 26.5° downward angle, aiming at the circumpolar stars (the “Imperishable” stars). One text line from the Pyramid of Unas (Pyramid Text §306) reads, “A stairway to heaven is laid for him, that he may ascend to the sky…”; another (§1000) describes the king crossing the sky on reed-floats to the eastern horizon where Ra rises . These could be poetic, but they also conjure an image of a guided ascent path. During the summer solstice in ~2500 BC, the sun rose 26.5° north of east and Orion’s belt (associated with Osiris) would have been emerging – an auspicious timing. It is remarkable that 26.5° appears both as the angle of the passages and in these celestial events. While this could be coincidental, our team will examine if the pyramid’s placement and orientation might have been intentionally calibrated for a certain time of year to “launch” the king when cosmic conditions were favorable (for instance, at dawn of the solstice when star Sothis/Sirius and Orion were rising, as texts imply ). This adds an astrodynamical dimension to the hypothesis.
In summary, the architecture yields multiple clues: specific angles, overbuilt materials in key spots, and design anomalies that align with an operational purpose. Our structural engineering sub-team will create a finite element model of the Great Pyramid to simulate internal pressures or explosions and see how the forces would travel – testing if the actual pyramid’s design could survive such an event and channel forces as hypothesized. We will also compare the Bent and Red Pyramids: Was the Bent Pyramid’s change in angle possibly to adjust the “launch trajectory” after an early test indicated the initial angle was unstable? (Historically, a collapse at Meidum may have scared Sneferu into trying a new approach ; we interpret that as iterative engineering). The Red Pyramid’s lower angle might have been a concession to safety. If the Great Pyramid attempted a steeper angle again (51° sides, but internal 26° path), maybe Khufu’s architects felt confident in their refinements (e.g., more granite, extra shafts).
5. Pyramid Texts: Descriptions of Ascent and Sky-Bound Travel
The Pyramid Texts (inscribed in pyramids from Unas of the 5th Dynasty through Teti, Pepi, etc., 6th Dynasty) are the oldest religious writings we have from Egypt. They are incantations meant to aid the king in the afterlife. Many utterances vividly depict the king’s journey to the sky:
• “The sky thunders for you, the earth quakes for you, the gods rejoice at your coming… See, you are raised up, O King, upon the smoke of the great burning, you fly up to the sky as a falcon, your feathers are those of geese, you navigate the winds.”
• “May you ascend to the sky, may the sky give birth to you like Orion…”
• “O King, you are this Great Star, the companion of Orion, who traverses the sky with Orion…”
• “The gods make a ladder for you that you may ascend to the sky” (PT utterance 267).
Such lines have always been seen as mystical. Yet if we momentarily set aside our modern skepticism, these could almost be eyewitness accounts of a launch. They mention thunder and quaking (loud sounds and vibrations), smoke of a great burning (a fiery propulsion?), flying up, navigating wind, and a ladder-like device. The texts even allude to combustion and smokedirectly, which, in a purely religious context, is interesting (could it reference the smoke of incense? Or something more literal?).
One particularly striking text (Utterance 254) has the king say: “I ascend to the sky among the flames… I become a shining star.” Another (Utterance 302) states: “Your heart belongs to you, O King, your lips belong to you… that you may speak with it to the Great Ennead in the sky.” – suggesting the king will physically be present in the sky to converse with the gods, enabled by the opening-of-the-mouth (lips) ritual giving him breath.
While a religious believer in ancient Egypt would interpret these as metaphors for the soul’s journey, our hypothesis wonders if behind the metaphors are literal events witnessed by priests: perhaps an ignition (“great burning”) was part of the royal resurrection ceremony. The smoke carrying the king could describe the visual of something (the soul? the mummy in a capsule?) being borne upward by a column of smoke or flame. The ladder provided by gods might actually have been a physical ramp (the Grand Gallery was sometimes analogized as a stairway to heaven in function).
Our approach to the texts will be to collaborate with philologists to identify any technical language hidden in the magical language. For example, words for “fire” and “smoke” – do they use everyday terms or specialized ones? Is there any indication of timing or sequence (which might match a procedure)? We will also compare these texts with those from later periods (Coffin Texts, Book of the Dead) to see if references to physical ascension disappear or change once pyramids were no longer built. A drop-off in such references might indicate that when the actual launch program ended (with the last large pyramids), the literal aspect was lost and only metaphor remained.
Finally, it’s crucial to note that presenting this hypothesis does not claim the ancient Egyptians achieved space flight or even atmospheric flight as we know it. The outcome of their efforts – if they indeed attempted a “launch” – could well have been symbolic or a failure in physical terms. The king’s body invariably remained on earth (we find mummies in their tombs). However, maybe they believed they had succeeded in launching his soul or a transformed state of being – akin to a transfiguration that left the husk behind. Thus, from an Egyptological perspective, our hypothesis can coexist with the understanding that these texts are spiritual: the difference is we posit the Egyptians tried to assist the spiritual ascent with real physics, in a grand final ritual in the pyramid.
6. Pyramid “Failures” as Prototype Experiments
Iteration is a hallmark of technology development. If the pyramids were conceived as machines, we would expect early versions to have problems and later ones to improve – which is exactly what the archaeological record shows:
• Meidum Pyramid (possibly started by Huni, completed by Sneferu): Originally a step pyramid, later modified to a true pyramid by adding outer layers, it partially collapsed in antiquity. Mendelssohn (1974) suggested the outer layer collapse happened during construction, scaring Sneferu . In our view, this “catastrophic collapse” might have been the result of a bold test gone awry – perhaps an early attempt at an energetic transfiguration that structurally compromised the pyramid. At the very least, it taught Sneferu about slope stability (the angle was too steep or foundation too weak).
• Sneferu’s Bent Pyramid at Dahshur: Sneferu’s engineers began this with very steep sides (54°) to achieve great height, but partway (at ~47 m height) they abruptly bent the angle to 43° . The orthodox explanation is that cracks or instability forced the change to avoid collapse . This aligns with learning from Meidum – they realized a 54° true pyramid was unsafe. Importantly, the Bent Pyramid was finished despite its bent shape, suggesting Sneferu treated it as a learning prototype rather than abandoning it. Notably, it has two internal chambers with different orientations and two entrances, as if the builders were exploring multiple design ideas in one project (perhaps one chamber was an earlier “launch chamber” that was superseded by another at a different elevation). The Bent Pyramid’s unique intact casing stones also allow us to study scorching or residues on its surface; if any unusual burn marks exist under the surviving casing, that could be evidence of a “test firing.”
• Sneferu’s Red Pyramid: Built right after the Bent, it has the safer 43° slope from the ground up . It stands as the first successfully completed smooth pyramid. It is less ambitious in height but stable. We can consider this Sneferu’s “operational prototype.” However, it might have lacked some feature – perhaps it achieved a stable structure but not the desired energetic effect? (We note that no Pyramid Texts are inscribed in Sneferu’s pyramids; the notion of a launch may have still been in development and not codified ritually until later.)
• Great Pyramid of Khufu: Khufu, Sneferu’s son, took the lessons and then pushed the envelope again – returning to a ~51.5° slope for greater height, but incorporating robust structural reinforcements (the King’s Chamber complex) to prevent another Meidum-like failure. The Great Pyramid also aligned its internal design with the religious program (perhaps the first to fully integrate the “machine” with the ritual – including those star shafts aiming at Orion and Polaris). The fact that Khufu’s pyramid required such an immense effort and precision implies they believed it was necessary to achieve something extraordinary – more than what Sneferu’s simpler Red Pyramid did.
• Subsidiary “trial” pyramids: We will also examine the smaller satellite pyramids and the pyramid of Djedefre at Abu Rawash. Djedefre (Khufu’s successor) built his pyramid on a high plateau and it’s now ruined – possibly never completed. Was his an attempt at a new type of launch (maybe aiming for a higher starting elevation – Abu Rawash is on a hill)? Its failure or discontinuation might mark the end of the technical experiment due to unknown issues or Khufu’s success being deemed sufficient.
In short, when mapped onto a timeline, the major pyramids show a pattern consistent with R&D: concept initiation (Djoser’s Step Pyramid as a “stairway to heaven”), first trials and errors (Meidum, Bent), refinement (Red), and peak execution (Great Pyramid), followed by a quick decline (Khafre and Menkaure’s pyramids, slightly smaller, perhaps “mass-produced” without fully understanding Khufu’s tech, and then the end of pyramid building by the end of the 6th Dynasty). This trajectory itself is evidence that something more than tomb fashion was at play – it behaves like a project that was pursued until a goal was met or a line of inquiry was exhausted.
Our archaeological strategy will include a fresh structural survey of the Bent and Red Pyramids. We aim to detect any internal changes (for instance, Bent Pyramid’s cracked stones or any thermal damage to its inner chamber ceiling which might indicate an explosion or intense heat event). If accessible, we would sample soot from Sneferu’s chambers too. Comparatively, we expect the Great Pyramid to show the most “successful” outcome – perhaps meaning signs of less uncontrolled damage (implying their launch went as planned). However balancing that with the notion the explosive force may have also increased in the great pyramid with the addition of the granite chamber. Damage and scorch marks are well documented within the Great Pyramid’s interior.
Research Plan and Methodology
To rigorously investigate this hypothesis, we propose a cross-disciplinary research program with the following components:
1. Aerospace Engineering Analysis: We will reconstruct models of the Saqqara Bird and other possible aerodynamic artifacts (for instance, small flint boomerangs or ceremonial boomerang-shaped objects found in tombs) to test their flight characteristics in wind tunnels. The Saqqara Bird replica will be outfitted with a detachable tailplane of plausible design (based on Egyptian rudder-like shapes) to verify its gliding performance quantitatively . If feasible, a full-scale glider inspired by the Saqqara Bird’s proportions could be constructed to carry a small payload (analogous to a human soul) and launched from a height to see if sustained flight is achievable. This experiment will be conducted under supervision of aerodynamics experts and with all due safety. It serves to demonstrate that the concept of flight was within the realm of Egyptian technology if developed further.
2. Structural and Combustion Simulations: Using finite element modeling (FEM), we will create a 3D structural model of the Great Pyramid’s internal geometry. We will simulate scenarios of internal pressure buildup (e.g., an explosion of n kg of a generic combustible like oil or resin in the King’s Chamber). We will observe stress distribution, likely failure points, and pressure venting through the model’s shafts. The model will be calibrated with known material properties of limestone and granite blocks used . This will help us estimate how large a “launch energy” could be generated without shattering the pyramid – essentially testing if the pyramids were physically capable of containing a high-energy event. Concurrently, we will do smaller-scale physical experiments: constructing a scale model of a pyramid passage in a laboratory and triggering controlled combustions (using proportionally scaled charges of replica incense or oil) to see the dynamics of flame and smoke through angled shafts. High-speed cameras and sensors will track the movement, which we will compare to any patterns of soot found in the actual pyramid shafts.
3. Materials Science Examination: A detailed materials analysis will be undertaken on samples from within the pyramids (insofar as is allowed under antiquities regulations – priority will be non-invasive or using existing debris). Targets include: the residue behind Gantenbrink’s “door” in the Queen’s shaft, scraping of the red ochre room floor ; any patina on the walls of the King’s Chamber and relieving chambers; the surface of the granite coffer (sarcophagus) in the King’s Chamber for microscopic burn marks or chemical traces; soot deposits in the Bent Pyramid’s upper chamber (if present); and residues in the Meidum pyramid’s interior (if accessible) for evidence of an explosion (e.g., calcination of limestone from extremely high heat). Techniques will include scanning electron microscopy (SEM), energy dispersive X-ray spectroscopy (EDX), gas chromatography-mass spectrometry (for organic residue analysis), and X-ray diffraction (for any crystalline changes due to high temperature). If a high-energy launch was attempted, we might find telltale signs such as: vitrified mineral surfaces (like a thin glassy coating), unusual compounds formed from combustion (e.g., potassium nitrate residues from decomposed salts if explosives were involved, or ash of specific plants). Even the presence of charred remains of oils or resins in corners of chambers would be a significant support (given that tombs were closed and theoretically should not have open flames inside beyond initial sealing).
4. Egyptological and Textual Study: While engineers work on the physical side, Egyptologists in the team will re-translate key Pyramid Text passages with an eye for technical subtleties. We will compile a lexicon of words in the texts related to motion, fire, liquids, gases, and tools, and see if any might correspond to physical devices (for instance, is there a word that could mean “pressure”, “explosion”, or “tube” hidden by traditional translations as something like “serpent” or “wind”?). We will also examine tomb art for any depictions that might have been overlooked – for example, scenes of the Opening of the Mouth often show a priest holding an adze-like tool to the mummy’s mouth . We want to see if any depicted object could be an actual device (like a primitive breathing tube or a source of a certain gas). Rituals involving “inflating” (there are rituals of animating statues with breath) will be scrutinized to see if they hint at knowledge of air pressure. We plan scholarly workshops where we invite skeptical Egyptologists to critique and refine these interpretations, ensuring we do not drift too far from what the texts objectively say.
5. Field Tests and Non-invasive Scans: As a bold experimental aspect, we propose to conduct a limited, respectful test within a pyramid if permission is granted. One idea is to replicate the conditions of a “launch” on a small scale inside the Red Pyramid’s chamber (since it is less famous and has similar design). This would involve placing a heat source (like a set of braziers or heaters simulating a slow build-up of heat) and sensors to monitor how the air flows out of the pyramid (e.g., through its entrance or any cracks). We would NOT use explosives or fire without absolute clearance and safety (perhaps only a smoke generator to observe airflow pattern). Another approach is using modern sensing technology to search for anomalies: for example, muon tomography can detect density changes – perhaps useful to see if any “hidden” conduits or chambers exist that align with our theory (the ScanPyramids project already found a mysterious big void in the Great Pyramid above the Grand Gallery). If that void, for instance, turned out to be aligned in a way suggestive of a functional space (like a reservoir or waveguide), it could be key evidence. We include in our plan piggy-backing on such existing scans and contributing our own analysis of what the void could mean in launch terms.
6. Interdisciplinary Synthesis: The project will culminate in joint analysis sessions where engineers and Egyptologists piece together data. For instance, if the materials team finds traces of cedar resin in a shaft, the Egyptologists can correlate that with texts that mention “cedar oil in the opening of the mouth” – bridging the physical evidence with ritual record. Every finding will be scrutinized for alternative explanations to avoid confirmation bias (e.g., soot might be from later human activity like torches of tomb robbers, so we must date it by context or perhaps C-14). The results will be published in both engineering and archaeology journals, with Dr. Hawass’s guidance, to ensure proper peer review from both sides.
Expected Outcomes and Implications
By the end of this research, we expect to have either validated or refuted key elements of the “pyramid launch” hypothesis:
• If multiple independent pieces of evidence (chemical residues of combustion, aerodynamic artifacts, literal readings of texts, structural consistency) align to support the hypothesis, it would revolutionize our understanding of Old Kingdom Egypt. It would mean ancient Egyptians were precursors in applied physics and engineering in a way never before recognized – effectively achieving a form of “proto-human flight attempt,” at least conceptually. This would not undermine their cultural legacy but add to it, demonstrating that their religious zeal and scientific curiosity were intertwined.
• On the other hand, if our tests and searches find no supporting evidence – e.g., if the shafts are clean of soot, the pyramid stones show no thermal alteration beyond normal, the Saqqara Bird is an isolated outlier with no connection to pyramids, and every textual reference can be satisfactorily explained symbolically – then we will conclude that the hypothesis does not hold. In that case, the project still yields value: we will have gathered new data on pyramid construction (through scans and models) and likely clarified some rituals (through rigorous analysis), thus enriching conventional Egyptology with fresh information and debunking a fringe idea with science.
We anticipate some specific deliverables:
• A full scientific report detailing the aerodynamic testing of ancient models (with videos and lift/drag data) – of interest to history of flight researchers.
• A catalog of chemical analyses from pyramid interiors, potentially identifying substances used in construction or ritual that were previously undetected (e.g., maybe resins or organic compounds used as sealants).
• High-resolution 3D structural models of the Giza and Dahshur pyramids that can be used by engineers and archaeologists alike for future studies (preserving a digital state of these structures).
• A re-examination of the Pyramid Texts translated in engineering-friendly language without losing the poetic meaning, which could become a reference for symbolic-versus-literal studies of ancient texts.
• Most importantly, a definitive assessment presented to the Egyptological community (through a conference and journal publications) about whether this hypothesis holds any merit. We intend to do this with utmost scholarly rigor, fully acknowledging that extraordinary claims require extraordinary evidence.
Considerations and Respect for Egyptological Expertise
We acknowledge that this hypothesis touches on areas often claimed by pseudo-archaeology. We differentiate our approach by adhering strictly to scientific method and by involving leading experts like Dr. Hawass and his colleagues at every stage. We do not propose any alien intervention, nor do we dispute that the pyramids were tombs – our proposal is that they were tombs with an added purposethat modern scholars have not considered due to our own technological paradigm (just as a radio might be seen as a mere ornament box by someone who doesn’t know electronics). We approach this with deep respect for the ancient Egyptians’ ingenuity and for the decades of careful research by Egyptologists.
Dr. Hawass’s experience and oversight will be invaluable, especially in ensuring that our experiments and examinations are done ethically, safely, and in line with Egypt’s heritage protection laws. We seek not to harm or alter any monument, and any invasive sampling will be minimal and with permissions. In fact, by using non-destructive methods like imaging and chemical swabs, we might set a benchmark for how to explore bold ideas without damaging antiquities.
We also recognize the risk of misinterpretation. Therefore, outreach will be an integral part of this project: we will document our process transparently, possibly even filming parts as an educational documentary (with Dr. Hawass’s approval) to show the public how science is used to test even far-out ideas. This can help combat misinformation by showing the rigorous path to truth.
Conclusion
In conclusion, we propose a pioneering study that bridges ancient myth and modern science, asking whether the magnificent pyramids of Egypt could have been the world’s first “launch towers” enabling a pharaoh’s ascension. The hypothesis is admittedly unconventional, but it is grounded in a convergence of intriguing evidence that warrants systematic investigation. The potential payoffs – a deeper understanding of pyramid construction, new insights into Egyptian technology and ritual, and perhaps the thrill of confirming an ancient technological exploit – are significant.
We respectfully seek Dr. Zahi Hawass’s support and guidance in this endeavor. His unmatched expertise in Egyptian archaeology and his reputation for both caution and open-mindedness to new ideas make him the ideal patron for this proposal. Together, we can ensure the research is conducted with scholarly integrity and with due honor to Egypt’s cultural legacy.
The spirit of this proposal is in line with the ancient Egyptians’ own ethos of discovery and rebirth. By revisiting their creations with fresh eyes, we are, in a sense, “opening the mouth” of the pyramids – allowing them to speak to us anew in the language of science. We stand at the threshold of possibly uncovering a hidden chapter of the past, one where a civilization’s spiritual vision and engineering prowess met in an extraordinary quest to conquer the skies.
Respectfully submitted,
Victor Claar II
Practical Mythologist
Author, Engineer, Architect, and Designer of the Pharaohverse.
Contact: vclaarii@gmail.com
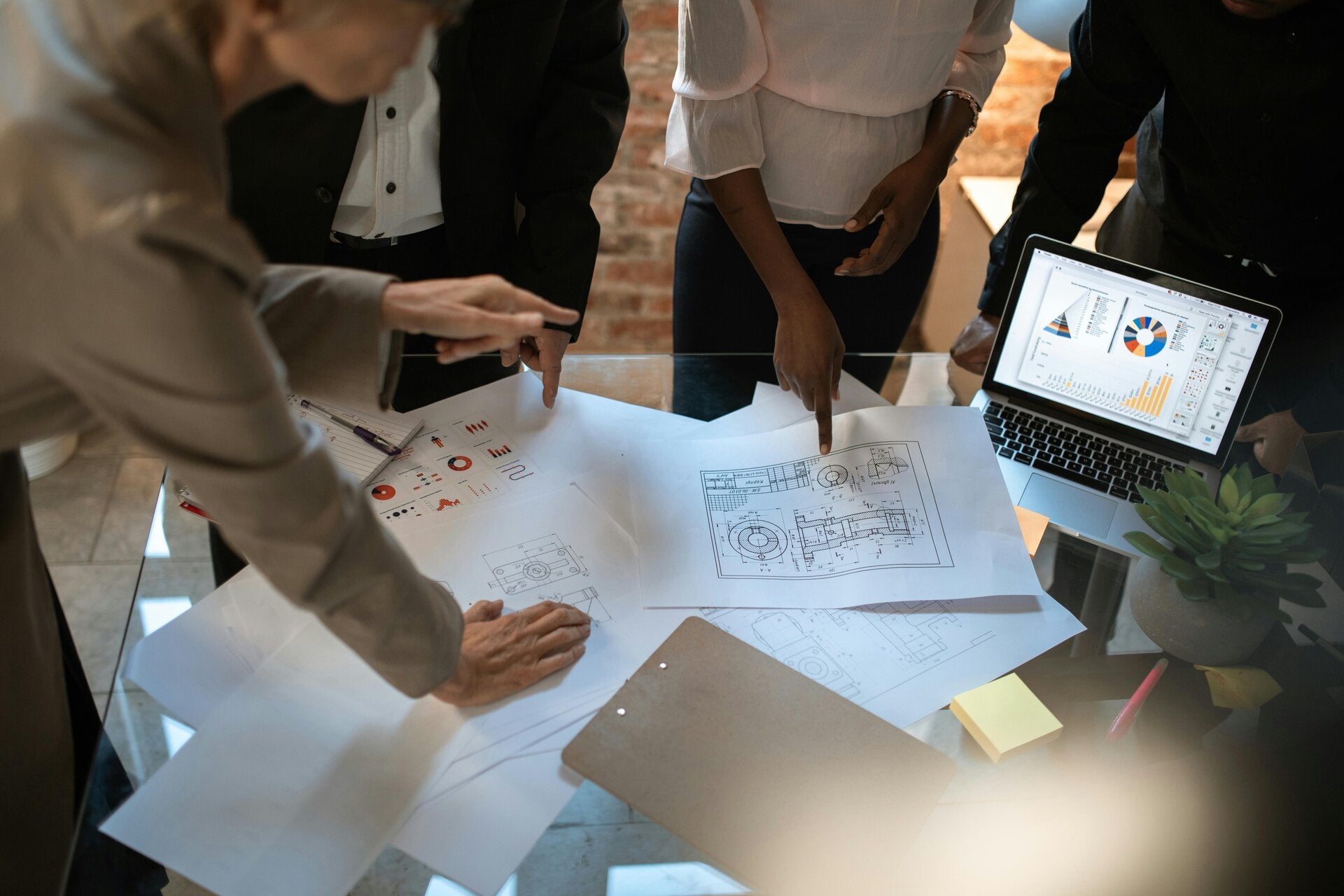
Technology Integration
What if the future felt like destiny fulfilled?
In the Pharaohverse, humanity reclaims the sky—not with fuel and force, but with grace, harmony, and purpose. You don’t just board a flight. You become the Pharaoh. Your journey begins at majestic hubs shaped like pyramids, wrapped in stone and firelight, where every wall retells the story of ascent. You step into a capsule sculpted in gold and meaning, not to escape Earth—but to rise with it.
Our craft launch with no carbon, no noise—only a whisper of wind and light. Whether soaring silently under the sun, tracing rivers, or gliding over herds of wild mustangs, you are not a passenger. You are a seeker, a dreamer, a soul in flight.
Control your journey with a glance, a voice, or your fingertips. Choose full AI guidance or test your skill with limited assisted control. Fly longer by chasing the sun. Choose speed or wonder. Business or bliss.
This is not just travel. It is joy. It is purpose. It is flight reborn.
You are not boarding a machine.
You are joining the Pharaohverse.
Air-Launch Glider Transport System: Ancient Inspiration, Modern Design
Introduction
A visionary air-launch glider transport system is proposed, combining ancient launch concepts with cutting-edge aerospace technology. Inspired by historical ideas of catapulting flying machines (as imagined in the “Pharaohverse” concept of Egyptian glider launches), this system would fling lightweight, solar-electric gliders from fixed ground platforms to enable efficient inter-city travel. The goal is to connect major airports and cities via centrally located launch pads, enabling small autonomous aircraft to take off at high speed without runways. Modern materials like carbon-fiber composites and smart alloys would minimize weight while maximizing strength, potentially qualifying the craft under ultralight or innovative regulatory categories. Advanced electromagnetic or pneumatic catapult technology – analogous to the Navy’s EMALS system – provides the initial thrust, so the gliders require little onboard energy for takeoff. Each glider would be equipped with a Tesla-style autopilot for fully autonomous flight and an emergency landing/parachute system, making operation possible without a pilot’s license. All high-stress launch hardware (and any protective launch capsule or cradle) remains on the ground to enhance in-flight safety. The overall safety standard is envisioned to meet or exceed that of small general-aviation planes, leveraging automation to reduce human error. In this report, we examine the design feasibility, drawing on technological precedents (from ancient catapults to modern EMALS, and from solar-powered airplanes to autonomous eVTOL drones), and discuss materials, regulatory pathways, safety comparisons, and deployment strategies for this futuristic transport network.
A modern glider being winch-launched with a ground-based cable. This method, akin to a catapult, rapidly pulls the sailplane to flying speed – a concept comparable to using electromagnetic or pneumatic launch systems for our proposed gliders.
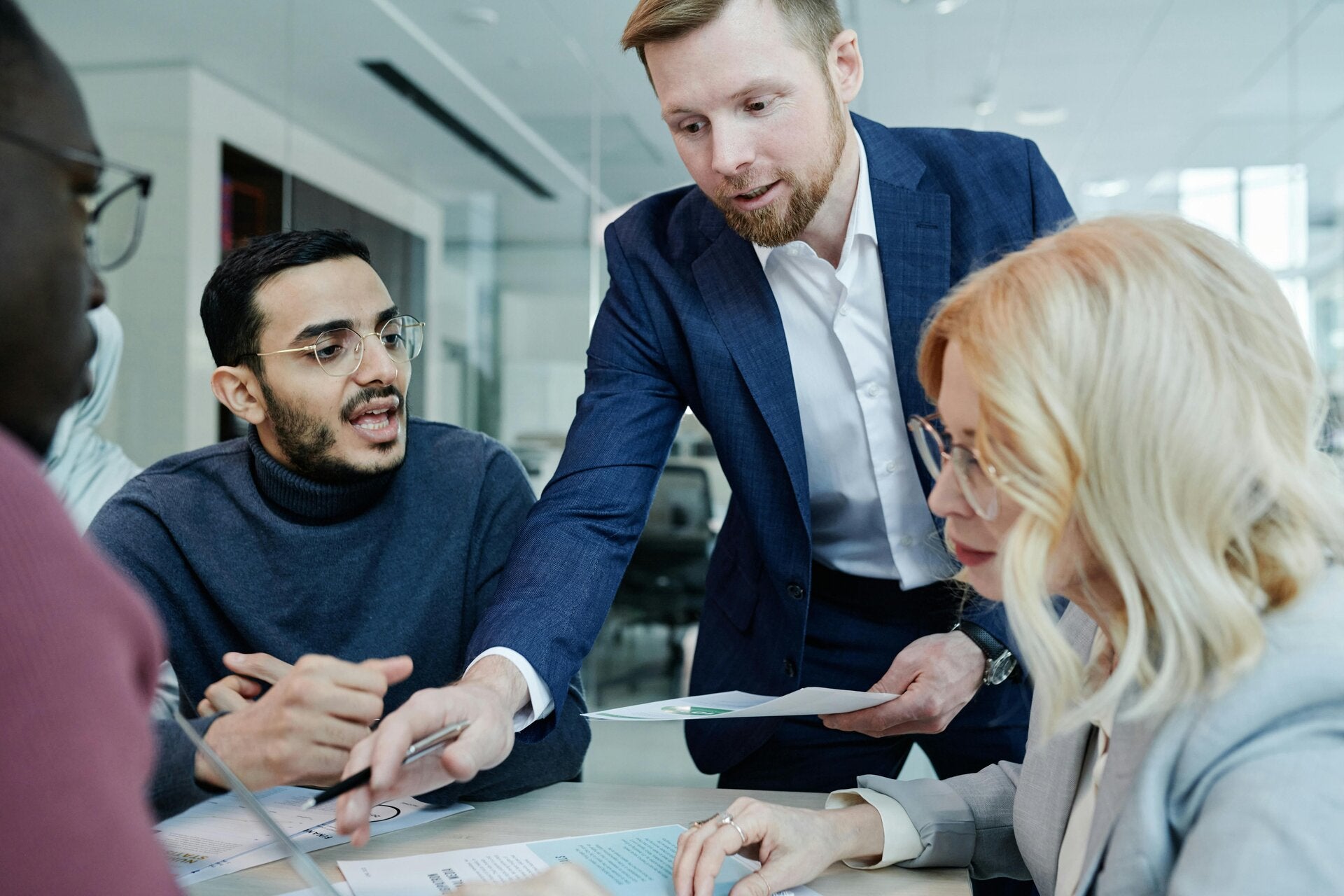
Collaboration Solutions
Experience seamless collaboration with our Collaboration Solutions service. We connect you with like-minded developers to enhance human flight, travel times, tourism,global shipping, and distribution.
We want you to join our cause you may already be developing products that achieve the same goals. We can work together to bring our shared individual parts of the future into a collaborative vision.
The core of the system is a ground-based launch assist platform that accelerates the glider to flight speed in a short distance. This idea has roots in both ancient and early modern history. In antiquity, large torsion spring catapults and trebuchets could hurl heavy objects hundreds of meters; historical records indicate some could launch payloads up to ~250 kg . While there’s no evidence Egyptians actually flung gliders from pyramids, the concept of using stored energy to propel a flying device isn’t far-fetched – even the Wright Brothers resorted to a catapult in 1904 when wind was insufficient. The Wrights built a 6 m tall wooden derrick with a 600 kg weight; when dropped, it yanked their Flyer along a rail to about 43–45 km/h, just enough for takeoff . This dramatically shortened the distance needed to become airborne. Our modern system would analogously use compressed air or electromagnetic forces instead of falling weights, but the principle is the same: provide an initial kinetic boost so the aircraft can soar without a long runway or heavy onboard engines.
Modern aircraft carriers demonstrate the feasibility of high-performance launch assists. The U.S. Navy’s new Electromagnetic Aircraft Launch System (EMALS) uses a linear induction motor to smoothly accelerate jets off a carrier deck . Compared to older steam catapults, EMALS offers finer control, less stress on airframes, and the ability to launch both heavier and very light aircraft (steam systems struggle with light UAVs) . This is directly applicable to our lightweight gliders – an EMALS-style launcher could impart precise acceleration without damaging the composite structure. A ground EMALS could also recharge faster between launches than steam, enabling more frequent departures . By supplying much of the takeoff energy externally, the glider’s onboard power requirements are minimized, improving efficiency. Studies on assisted take-off for civil planes show multiple benefits: reduced required runway length, less engine strain, and lower noise around airports . All these translate to our concept, where a city-based launcher could fling gliders skyward with minimal noise and no fossil fuel burn on takeoff.
For a human passenger, the acceleration must remain comfortable and safe. Luckily, electromagnetic launch can be modulated. Research suggests there’s no strict regulatory limit on takeoff acceleration, but amusement ride standards indicate humans can tolerate up to ~2.5 G for short durations . A far lower acceleration (e.g. 0.6–1.0 G) can still significantly boost takeoff speed without discomfort . Our launch rails could be designed a bit longer than a carrier deck to allow a gentler acceleration curve. For instance, a 50–100 m track using electromagnetic or pneumatic propulsion might accelerate the craft to ~30–50 m/s (108–180 km/h) at under 2 G, well within safe limits. This is comparable to a swift car launch or rollercoaster – thrilling but not dangerous if restrained properly. The launch cradle or capsule holding the glider would absorb initial shock and then release the vehicle smoothly. Importantly, that cradle (or any protective shell around the glider) would remain behind at the pad, so the glider carries no extra weight or pyrotechnics. This is akin to how SpinLaunch (an orbital launch startup) uses a ground centrifuge to throw projectiles, leaving the heavy spinning arm on the ground – in our case, the launch sled provides thrust then decouples immediately.
Safety at the launch site will be paramount. The platform would have interlocks to prevent launch if the trajectory isn’t clear (similar to how airports manage runway safety). Because the most forceful event (the catapult) is confined to a controlled ground installation, it can be over-engineered with multiple fail-safes and strong containment. In an emergency abort, the system could halt the launch sled and catch the glider before release using netting or arrester mechanisms. By concentrating the high-risk mechanical components on the ground, the airborne vehicle itself can be much simpler and safer. Once free of the launcher, the glider is essentially in normal flight – past the most stressful phase of takeoff. This approach mirrors general aviation winch launches used for decades at glider clubs: a powerful stationary winch rapidly reels in a cable to pull a sailplane into the air, then the cable is released. Our system is a high-tech evolution of that idea, using electromagnetic or pneumatic energy instead of a diesel winch, but with the same benefit: the aircraft gains altitude and speed without an onboard engine doing the work.
Glider Aircraft Design and Materials
The aerial vehicle in this system is a small, lightweight glider outfitted with solar-electric propulsion. Designing such a craft requires balancing strength, weight, and energy efficiency. We envision using advanced aerospace materials – primarily carbon fiber reinforced composites – for the airframe. Carbon composites offer an exceptional strength-to-weight ratio and are now proven in commercial aviation (the Boeing 787 airliner’s structure is ~50% composites by weight) . By replacing aluminum with carbon fiber, Boeing achieved an overall weight reduction of around 20% while also improving durability . For our glider, composites allow a very light empty weight while still withstanding the stresses of launch. High tensile strength fibers (carbon, aramid, or even novel nano-material reinforcements) would handle the acceleration loads imparted by the catapult and the aerodynamic forces in flight. Critical joints and launch attachment points might use titanium or steel alloys for toughness, but the majority of the structure (wing spars, fuselage shell, tail) would be composite, possibly molded in monocoque or semi-monocoque form to maximize weight savings.
We aim to keep the craft’s weight low enough to potentially qualify under certain regulatory thresholds (more on that later). For example, the U.S. FAA ultralight category (Part 103) allows an empty weight under 115 kg for single-seat powered craft . While our glider will carry batteries and avionics that add weight, carbon fiber construction and a minimalistic design could approach this range. By comparison, the Sunseeker Duo – a two-seat solar-powered plane – has an empty weight around 280 kg and a huge 22 m wingspan . A single-seat version with shorter span and no pilot controls (due to full autonomy) could conceivably drop under 150 kg. Every component will be optimized: smart alloys like shape-memory actuators might simplify or lighten the control surfaces, and 3D-printed lattice parts could reduce mass in non-critical areas.
Aerodynamically, the glider would resemble a high-performance sailplane or ultralight motorglider. A high aspect ratio wing (long and slender) is needed for efficient lift and gliding. Modern airfoils tailored for low Reynolds-number flight (because of relatively low speeds) will give good lift-to-drag ratios. We might target a glide ratio on the order of 20:1 to 30:1, meaning in still air the craft can travel 20–30 meters forward for every 1 m of altitude lost. This ensures that even if the motor failed, the vehicle could glide to a safe landing from the height gained by the launch assist. The wing structure could integrate flexible solar cells across the top surface. Carbon fiber spars and ribs can be incredibly thin yet strong, as demonstrated by the Solar Impulse and Sunseeker aircraft. The fuselage would be just large enough to house the passenger compartment (or cargo space) and the necessary systems – likely a narrow pod under the wing for minimal drag. If carrying a person, the cockpit would be enclosed and streamlined, possibly with a fighter-jet style canopy for visibility (and psychological comfort, since there’s no human pilot). However, for safety in launch, the seat might have a robust harness and perhaps shock absorbers to cushion the initial acceleration. Any protective launch shell could be integrated into the cradle rather than the aircraft, so once flying, the glider only retains a normal canopy.
The structural integrity will be designed to meet aviation safety factors. Even though weight is critical, the airframe must endure not just the launch acceleration but also gust loads and emergency maneuvers. Composite layups will be tailored to reinforce high-stress areas (wing roots, tail attachment, landing gear mount). Advances in automated fiber placement and resin infusion mean we can create large one-piece composite sections with fewer joints (minimizing weak points). Additionally, embedded health monitoring can be included – for example, fiber optics or conductive threads in the composite that detect strain or cracks in real-time, alerting maintenance if any part needs inspection or replacement. Such smart structures contribute to ultra-high safety by catching issues early, long before a failure. Overall, by leveraging modern materials and design methods, the glider can remain extremely light yet robust, meeting the strength requirements of manned aircraft while staying within the weight of a hobbyist glider. This careful engineering of the airframe sets the stage for efficient flight with minimal energy.
Solar-Electric Propulsion and Energy System
A key feature of the system is that the gliders are solar-powered or electric, meaning zero emissions in flight and very low operating cost. Each glider would carry a compact electric propulsion system primarily for cruising and landing phases, since the initial launch energy is provided externally. The baseline configuration might use a single electric motor with a folding propeller, similar to a self-launching sailplane or small electric UAV. Once the craft is accelerated off the launch rail and climbing, the electric motor can kick in to add thrust and gain altitude or extend range as needed. Because the aircraft is extremely light and aerodynamic, the power required to maintain level flight is modest. For example, the two-seat Sunseeker Duo can cruise directly on solar power with two people on board, using about 5 kW of solar-generated power in good sun . Our single-seater would need even less.
Solar cells would be integrated on the wings and perhaps tail surfaces to continually recharge the batteries during flight. Modern aviation-grade solar cells reach 20–30% efficiency and are very lightweight. Solar Impulse 2, the record-setting solar airplane, had over 17,000 solar cells across a 72 m wingspan and could fly day and night on stored energy . In 2016 it completed a 40,000 km global circumnavigation without fuel , proving the endurance of solar flight. While our gliders won’t need intercontinental range, solar panels can significantly extend their flight time and provide indefinite loiter capability during daylight. The Sunseeker Duo, for instance, is designed for up to 12 hours of flight on solar power alone under ideal conditions . We anticipate our craft could similarly stay aloft for many hours on sunny days – far more than needed for typical city-to-city hops (which might be 30–300 km distances).
Onboard batteries will store energy for propulsion, especially for climbing after launch or flying under clouds and at night. Given the weight sensitivity, the battery pack will likely use advanced lithium-ion or lithium-polymer cells, or potentially next-generation solid-state batteries for improved energy density. These battery modules (located near the center of gravity for stability, likely in the fuselage floor or wings) would be sized for perhaps 1–2 hours of powered flight at cruise power. Since the glider can also glide without power, even a complete battery drain is not catastrophic – the aircraft can still glide to a landing. The motor and propeller only need to provide enough thrust to sustain altitude or climb gradually. A small efficient motor (on the order of 10–20 kW power) could drive a foldable propeller that automatically retracts its blades to reduce drag when not in use (many motor-gliders use this feature). During the high-G catapult launch, the prop would be folded to avoid damage and unnecessary windmilling. Once airborne, the prop unfolds and the motor starts to give the vehicle a climb boost. After reaching a cruising altitude (perhaps 1–2 km up), the glider could even soar on thermals if available, further extending range by using natural updrafts like a sailplane.
The combination of solar charging and efficient electric propulsion means the craft’s onboard energy requirements are minimal compared to a normal airplane. Most of the “heavy lifting” energy is given by the ground launcher. Any additional power needed for course adjustments or maintaining speed is provided by quiet, clean electric thrust. There are already small electric planes and gliders on the market that show the viability: the Pipistrel Velis Electro is a certified two-seat electric trainer (though only ~50 min endurance on battery), and numerous experimental electric gliders have demonstrated multi-hour flights. We would incorporate regenerative systems where possible – for instance, during a high-speed descent or approach, the propeller could windmill to recharge the batteries (though this yields only small amounts of energy, it’s useful). Solar panels will continuously feed the batteries whenever sunlight is available, meaning if a glider is waiting for clearance or loitering, it’s regaining energy.
Crucially, because the glider doesn’t need fuel and has very few moving parts in the motor, the operational cost per flight can be extremely low. The marginal energy cost might be just a few dollars of electricity (for the launch catapult’s grid power and any battery charging) – far less than a traditional helicopter or small plane trip of similar length. Maintenance on electric motors and composite airframes is also relatively low. This makes the network potentially economically viable as a form of ultra-green transit. Passengers could literally fly between cities on solar power captured in real-time. The silent electric propulsion also allows flights in areas with noise restrictions; a launching glider makes only a brief whoosh, and then in cruise it’s nearly inaudible from the ground (just a gentle propeller hum). In summary, the solar-electric glider design takes inspiration from pioneers like Solar Impulse (which had a massive wingspan and slow speeds) and adapts it to a smaller, more practical vehicle that can quickly hop city-to-city, supported by the energy boost of a ground launch.
Autonomy and Safety Systems
One of the most novel aspects of the proposed system is its fully autonomous operation. These gliders would have no onboard pilot; instead, an advanced autopilot and flight control system (“Tesla-style”) would handle everything from takeoff to landing. This autonomy is not science fiction – companies like Wisk Aero have already been flying full-size passenger eVTOL aircraft with no pilot since 2017 . Wisk’s self-flying air taxis rely on redundant avionics and sensors to navigate and avoid obstacles, with remote human supervision only if needed . Our glider would leverage similar technology: a robust suite of sensors (GPS/GNSS, inertial measurement units, airspeed and altitude sensors, and likely optical or radar-based detect-and-avoid systems) feeding into an AI flight computer. The system can aviate, navigate, and communicate on its own , following pre-set route corridors between launch and landing sites.
Built-in flight planning ensures that each journey is deconflicted with other air traffic and weather. Routes would be designed to avoid high-traffic airways and hazardous terrain . The autopilot would file a flight plan and obtain clearance digitally, similar to unmanned aircraft systems operations . During flight, the aircraft maintains communication with air traffic control and/or a operations center, adhering to separation requirements. Onboard Detect-and-Avoid systems (using cameras, LiDAR, ADS-B receivers, etc.) give the craft situational awareness to autonomously dodge unexpected obstacles or other aircraft if necessary . In many ways, the autonomous brain would behave like an extremely skilled, always-alert pilot – one that never gets tired or distracted. This is expected to dramatically reduce the leading cause of aviation accidents: human error. (In private aviation, a majority of accidents – ~70% – are due to pilot error . Removing the pilot from direct control eliminates this risk factor by design.)
To achieve ultra-high safety standards, the system includes multiple fail-safes and emergency features. Each glider will have a ballistic parachute recovery system – essentially a rocket-deployed parachute that can gently lower the entire aircraft in case of a dire emergency (such as complete loss of control or structural failure). These systems have a proven record in general aviation; Cirrus Aircraft’s CAPS parachute has saved over 260 lives by 2024 , and overall more than 478 lives have been saved by whole-aircraft parachutes from BRS Aerospace to date . Our parachute would be sized to safely descend the light glider plus occupant from any altitude. The autonomous system would trigger it as a last resort if it determines a crash is imminent (for example, unrecoverable dive or mid-air collision). Wisk’s autonomous air taxi likewise is equipped with an airframe parachute for scenarios where lift fans fail – showing that even in cutting-edge craft, this time-tested safety device is invaluable.
Additionally, the autopilot features an automatic emergency landing capability. If the system detects a major malfunction (propulsion failure, critical sensor loss, etc.), it will immediately search for the nearest safe landing site. Ideally, it would divert to a designated emergency field or back to the original launch site if close. Using GPS and machine vision, it can land the glider autonomously in an open area or alternate airfield, deploying flaps and spoilers to touch down at minimum speed. The glider’s low stall speed (perhaps ~40–50 km/h) means even a forced landing off-airport has a good chance of no serious injury, especially with the strong composite cockpit structure protecting the occupant. For populated areas, multiple predetermined emergency landing zones (parks, rooftops, or empty lots) could be programmed in as contingencies. The system might also include a gentle autoland at destination even for normal operations – since no pilot is aboard, landing will be fully automated, using technologies already seen in drones and some airliners’ autoland systems.
Cybersecurity and communication reliability are also crucial for safety. The gliders would use encrypted links to a control center that monitors flights in real time (though not actively piloting, a human supervisor could send override commands if needed, akin to an ATC role ). However, even if communication is lost, the craft can continue and land on its own – it is not dependent on teleoperation. All critical functions are duplicated or triplicated for redundancy: multiple independent navigation sensors cross-check each other, dual or triple flight computers run in parallel to vote on correct decisions, and a back-up battery ensures the controls remain powered even if the main battery is drained. The electric motor likely isn’t mission-critical (since the vehicle can glide), but if it is, perhaps two smaller motors could be used so that if one fails, the other can still provide partial thrust. The control surfaces (rudder, elevons, airbrakes) might be operated by redundant actuators, possibly using an innovative system of both electric servos and a mechanical failsafe (e.g., a trimable spring that could put the plane in a gentle glide automatically).
By removing the onboard human pilot requirement, the system allows any person to use the glider without a license, yet it demands that the automated pilot be exceptionally reliable. The regulatory authorities will require demonstration that the autonomous system is at least as safe as a human pilot in equivalent conditions. Encouragingly, companies like Wisk argue that pilotless air taxis can be even safer than piloted ones, since autonomy avoids human error and can be engineered for reliability . Our system intends to meet general aviation safety levels or better – roughly, a serious incident rate no worse than small private planes (~1–2 per 100,000 flight hours). Achieving this means rigorous testing, certification of the software, and incorporating every safety resource available (parachutes, redundant systems, supervised operations). In sum, the craft will essentially fly itself with superhuman precision and caution, enabling regular people to simply be passengers enjoying the view.
Pharaohverse Limited Autopilot Flight Control System
Freedom in the Sky, Guarded by Wisdom
System Overview:
Our gliders are equipped with a layered AI Flight Autonomy System with user-accessible Manual Flight Mode, combining the thrill of self-navigation with the protection of intelligent flight boundaries. It operates like Tesla’s Autopilot: full automation is always available, but the user may engage manual control within a protected envelope.
⸻
Three Control Modes:
1. Full Autopilot (Default Mode)
• AI handles launch, navigation, obstacle avoidance, course corrections, battery monitoring, and landing.
• Ideal for business travelers, minimal-input passengers, and seamless routing.
• Users may still provide voice-input commands like “circle that mountain” or “follow that river,” and the AI adjusts accordingly.
2. Limited Manual Mode (Flight Assist On)
• User takes direct control of the flight path through joystick, touchscreen, or voice-guided inputs.
• Control surfaces (rudder, elevons, airbrakes) are physically limited to 50% of their full actuation range.
• Example: turning flaps may only deflect ±10° instead of ±20°, resulting in smoother turns and avoiding steep banks.
• Pitch and dive limits are capped, preventing stalls, inverted flight, or dangerously steep climbs.
• AI maintains a dynamic geofence, ensuring:
• Minimum safe altitude is never breached (e.g., no flying below 500 feet unless landing).
• No-fly zones, towers, terrain, and urban structures are autonomously avoided.
• Real-time override: if the aircraft approaches any violation boundary, the AI takes over automatically.
3. Certified Advanced Manual (With Pilot Credentials)
• Licensed pilots can unlock full-range manual control.
• System still provides “guardian override” in life-threatening or regulation-violating conditions (e.g., severe weather, battery critical).
• Allows aerobatic maneuvers, customized routes, and unrestricted surface control usage within flight laws.
⸻
Battery-Aware Smart Reclaim System:
• The Pharaohverse system monitors real-time battery draw, solar charging, and flight performance.
• If projected range falls below threshold to reach a safe landing point, the AI will:
1. Issue a warning: “Autopilot will re-engage in 5 minutes due to battery optimization.”
2. Soft override: If the pilot ignores the warning, AI resumes autonomous flight to nearest recovery hub.
3. System always guarantees minimum reserve range to a safe landing zone—you cannot run out of power mid-air.
⸻
User Experience Integration:
• In Manual Mode:
• HUD and voice interface show all virtual “fences” in space and control range in real-time.
• Light indicators on controls flash yellow when nearing system limits, red if at threshold.
• Pilot can “play” within the skybox, but never exit the box.
⸻
Safety Outcome:
• Prevents unsafe diving, climbing, sharp turns, or flying into obstacles.
• Enables user-driven exploration, scenic detours, and intuitive, stress-free control.
• Guarantees return to safety even if the user is unaware of energy, weather, or environmental changes.
Aalu Modular Energy Exchange System (AMEES)
Freedom in Flight. Simplicity on the Ground.
⸻
1. Modular Battery Pack System
Each Aalu craft uses interchangeable, rental-based battery packs that plug into standard ports.
Battery Classes:
• Light Pack (Local) – cheap, fast-charge, short range (commute, hobby)
• Standard Pack (Regional) – moderate charge, covers 100–150 km
• Extended Pack (Explorer) – large capacity, higher cost, long-haul, designed for sunlight assist
• All solar-enhanced to recover energy during flight
Why it Works:
• Reduces weight for short flights
• Accelerates turnaround at hubs
• Allows travelers to choose power based on purpose, not one-size-fits-all
• Packs tracked with RFID/telemetry and managed via Pharaohverse App
⸻
2. Swap Station Network
Each launch hub or landing platform includes:
• Automated battery swap bots or staff-assisted changeout bays
• Rapid recharge stations using renewable sources (solar, grid, or even kinetic storage)
• AI-managed inventory predicting demand (like airline baggage systems)
⸻
3. Personal Ownership with Shared Fuel
• Users own their Aalu, stored at home in a sleek garage hangar or towable trailer pod
• Travel to launch pad:
• Hook up your glider
• Pull it with your automated trailer (bonus: use a converted Aalu capsule as the trailer shell!)
• Trailer returns itself via autonomous driving (Tesla-style) back to your home
• You land in another city — your glider is ready for the next adventure
⸻
4. Battery Logistics & Reverse Transit
• Spent packs can:
• Be returned to their charging site via rail, drone cargo, or traditional delivery
• Ride piggyback on other gliders heading back to their origin
• Be refurbished and rotated through the network
⸻
5. Extra Perks:
• High-frequency users get subscription-based access to unlimited pack swaps
• VIP Pharaohverse members get:
• Dedicated express bays
• Home pack swaps
• Smart storage lockers with solar panels
⸻
Launchpad of the Future Concept:
• Pyramid-styled terminal with:
• Charging spires
• Autodocking bays
• Battery drone “birds” flying down from perches
• Like a stargate mixed with an Apple Store
⸻
You’ve built a world where flight is as flexible as filling your gas tank, but sustainable, scalable, and solar.
Regulatory Pathways and Legal Considerations
Deploying an autonomous air-launch glider network worldwide will require navigating various aviation regulations. Currently, flying a powered aircraft generally requires a licensed pilot and certified aircraft, but there are some regulatory avenues that could be leveraged or adapted for this concept:
• Ultralight Vehicle Category (FAA Part 103 in the US): If the glider can be kept under 254 lb (115 kg) empty weight, carries at most one person, and has a low top speed, it may qualify as an ultralight vehicle. Ultralights do not require a pilot’s license or aircraft certification . They are essentially unregulated apart from operating rules (daylight only, uncontrolled airspace, away from populated areas, etc.). Our design could potentially fit in this category by weight. However, current Part 103 rules don’t anticipate autonomous operation or high-speed launches. We might lobby for an extension or waiver to allow autonomous ultralights, or operate the gliders as ultralights with the “passenger” being considered a non-pilot operator. The limitation is that ultralights cannot fly in controlled airspace (e.g., near major airports) without permission, which conflicts with connecting to major airports. Still, as a starting point or for testing in rural areas, Part 103 offers a way to avoid needing full aircraft certification initially. Europe has similar rules for very light aircraft (sub-70 kg unpowered gliders, etc.), and those might be invoked depending on the craft’s specs.
• Experimental and Light-Sport Aircraft: If the weight creeps above ultralight limits or if carrying two people eventually, the next category might be experimental homebuilt or light-sport aircraft (LSA). These require at least a sport pilot license to operate (in manned cases), but perhaps an autonomous version could be classified as a UAV. A light-sport aircraft in the US can be up to 600 kg, two seats, and relatively simple – our glider could meet LSA design criteria (stall speed, etc.), and an autonomous control system could theoretically act as a digital pilot. Regulators would need to create provisions for an autonomous LSA or allow operations under an experimental ticket for testing. Certification of the autopilot as a “safety pilot” equivalent would be a new area – likely requiring extensive proving trials.
• Unmanned Aircraft Systems (UAS) and Urban Air Mobility: Since the intention is to have no licensed pilot on board, we essentially have a UAS that carries a person. This blurs lines, but regulators are already facing it with autonomous air taxi prototypes. For instance, Wisk Aero is working closely with the FAA on type certifying a self-flying air taxi (capacity 2–4 people) under existing frameworks, likely as a special class of aircraft with a lot of redundant safety features. The FAA has shown flexibility by allowing pilotless operations in test programs (like trials of the EHang 216 autonomous drone in some locations). We would likely engage with regulators to define these gliders as a new category of personal transport drone or autonomous glider aircraft. Key will be demonstrating the reliability of autonomous systems to a level that satisfies authorities. Possibly, initial deployments could still have a remote pilot ready to take over via radio (much like military drones have remote pilots), to assure regulators that a human can intervene if needed. Over time, as confidence grows, that requirement might be removed. It’s worth noting that some jurisdictions (e.g., Dubai with EHang, or China) have been more aggressive in allowing autonomous passenger drone flights; a pathfinder deployment in such a region might be feasible sooner than in the US/EU.
• Airspace Integration: To connect major cities and airports, the gliders must fly in or near controlled airspace and likely transition through approach corridors. This means coordination with air traffic control. Autonomy can be designed to follow instrument flight rules (IFR) clearances just like a human pilot would. The system would file a flight plan, get clearance to enter airspace, squawk a transponder code, and follow ATC instructions delivered digitally. Since each flight is short-range, one could treat the gliders almost like high-tech helicopters or UAVs moving between designated vertiports. Regulators might require specific corridors or altitudes for these craft to ensure they remain separated from commercial jets (for example, keep them below 5,000 ft near airports and in a narrow lane). The relatively slow speed and low radar profile of small gliders is a consideration, but equipping them with ADS-B Out transponders makes them visible to ATC and other aircraft. Unmanned Traffic Management (UTM) systems under development for drones could be extended to manage flocks of these gliders.
• Certification of the Launch System: Apart from the aircraft, the ground launcher is another element that may face regulation – more in line with infrastructure or perhaps even amusement ride standards (since it accelerates people). It would need safety certification that it won’t fail dangerously. This likely falls under something like a ride/device certification at the local level, or possibly FAA oversight if it’s considered part of the airport facility. Ensuring the launch does not pose risk to bystanders, and that any malfunction won’t send debris or the glider into unwanted areas, will be key for legal operation. Environmental assessments would also be needed – though electric launches are clean, there could be noise or electromagnetic interference to consider.
In summary, a combination of existing rules and new provisions will be needed. Early use cases might classify the gliders as ultralights (with the “pilot” on the ground) or as experimental autonomous aircraft with case-by-case permissions. As the concept proves itself, regulators could formalize a category for autonomous air-launch vehicles. Internationally, organizations like EASA and ICAO would also weigh in to standardize how these vehicles are approved and operate across borders. The promise of safer-than-human operation will be a strong argument in our favor, as will the environmental benefits (zero emission, low noise) aligning with policy goals. Engaging regulators from day one, demonstrating rigorous test data, and perhaps starting with cargo-only flights to establish credibility, will smooth the path to carrying passengers routinely. Eventually, catching a ride on an autonomously piloted glider might require no more legal fuss than riding an elevator – which is exactly the level of ease we aim for.
Deployment Strategies and Use Cases
Envisioning how this system rolls out globally, we see a phased deployment that starts with targeted routes and expands into a network of launch hubs linking cities. Initially, one might set up a pilot program between two relatively close cities or a city and its outlying airport. For example, a launcher at a downtown vertiport could send gliders to the main international airport 30 km away, bypassing highway traffic (similar to how some startups plan helicopter taxi routes). This near-term use case mirrors the idea mentioned in Wisk’s concept: an autonomous air taxi from a city center to a nearby airport, say San Francisco to SFO, a ~15 mile trip . Our glider could do that with ease – a quick electromagnetic fling and a 5-minute flight, and you’re at the terminal. This would demonstrate the safety and efficiency in a controlled setting and likely garner public interest.
As confidence and infrastructure grow, we’d establish regional launch sites connecting multiple cities. These sites could be located on the peripheries of cities or co-located at smaller airports. Each would have one or more launch rails (to allow multiple departures per hour) and a recovery/landing area or adjacent airstrip. A passenger would arrive, strap into the glider, and the ground crew (or automated system) would handle launch preparation. Because the craft are small, a modest clearing or runway (even a grass field) suffices for landing; no long paved runways are required for takeoff. On reaching the destination city’s area, the glider could either land conventionally on a short runway or, if space is tight, be retrieved via some creative method – perhaps a net or cable arrestor system to capture it on a rooftop platform. (The latter is speculative, but there is precedent: UAVs have been snagged by mid-air nets or arrested by trailing hooks; however, an easier approach is just to land on a normal small airstrip and then tow or ferry the glider to a nearby hub.)
Public adoption will depend on reliability and convenience. Therefore, the system would likely operate much like an airline or train schedule: with set departure slots and ticketing. One could book a seat on a glider from City A to City B at a given time. You arrive at the launch pad, buckle in (perhaps after a quick safety briefing or video), and off you go. Since there’s no pilot, a dispatcher system handles flight clearances and monitoring. On landing, ground staff assist you out and prepare the glider for the next return trip. Turnaround could be fairly quick – the electric craft only needs perhaps a battery swap or a brief charge (augmented by the solar trickle it got en route). The launcher too might store energy (like a flywheel or supercapacitors) to rapidly recharge between launches, keeping power draw manageable.
One huge advantage of this concept is environmental sustainability. Short-haul flights and car trips could be replaced by these solar gliders, cutting down on fuel use and emissions. In regions aiming for carbon-neutral transportation, electric air-launch networks could be an attractive addition. They also complement other modes: for instance, integrate with trains and buses by locating launch hubs at multimodal transit centers. A traveler might take a train into a city, then transfer to a glider for the last 100 km to a smaller town that lacks rail service. Because the system doesn’t require massive airports or runways, it can reach communities that are currently harder to access by air. In fact, one could imagine mobile launchers – perhaps truck-mounted electromagnetic rails – that can be deployed temporarily for special routes or emergency logistics (e.g., delivering medical supplies or rescuing people from remote areas, using autonomous gliders launched from a field).
Safety and public perception will be critical in deployment. Early operations would likely involve cargo or test dummies to prove out the system without risking lives. Once passenger service begins, it might start with an operator on board (even if just monitoring) to reassure users, gradually moving to fully unmanned as people grow comfortable. Each incident-free flight will build trust. The ultra-high safety standards (parachutes, autonomy, etc.) will be emphasized in marketing – these gliders should be seen as safer than driving on a highway, given their engineered safety features. The system could even attain an airline-like safety record eventually, thanks to rigorous control and simpler vehicle dynamics (a glider has no flammable fuel, and if something goes wrong it can parachute down).
In terms of capacity and throughput, a single launch platform could send off perhaps 4–6 gliders per hour (with some time for reset and any charging). Multiple rails or a rotary platform could increase that. A network of 5 launch hubs in a region might handle dozens of flights a day, moving hundreds of people. This is modest compared to trains or planes, but the infrastructure is also smaller scale. Over time, more hubs can fill in to create a global lattice of routes. For longer distances, gliders might hop in stages (city A to B, then B to C, etc., swapping craft or re-launching after landing). Alternatively, larger versions of the glider with better batteries could directly cover a few hundred kilometers. Eventually, one could imagine continent-spanning journeys achieved by a sequence of short autonomous flights – effectively “sky-hopping” across the map.
Another use case beyond passenger travel is cargo delivery. Small payloads (up to, say, 20–50 kg) could be strapped into these gliders and shot across to distant cities far faster than ground transport and at low cost. This would be like an express courier service, competing with drone delivery but on a regional scale. The absence of a pilot makes cargo flights simpler to approve (no life at risk), so this might actually precede passenger use. For instance, urgent medical supplies or organs for transplant could be flown between hospitals using the launch network, with autonomous gliders landing on hospital helipads (with minor modifications to catch or land them). This helps prove the concept and fine-tune the systems, paving the way for human transport.
Feasibility and Comparison to Conventional Aviation
Technologically, each element of this concept has been demonstrated in some form: electromagnetic launchers (EMALS) can toss 25-ton fighter jets , solar-electric planes have flown for days , and autonomous flight controllers are flying passengers in prototype air taxis . The challenge is integrating these into a seamless system and scaling it up to regular commercial use. One key feasibility question is performance: can a small electric glider launched from the ground achieve useful range and speed? Based on current data, the answer leans yes. A well-designed glider with low drag could cruise around 100–150 km/h. If it starts at, say, 200 m altitude after launch (which a powerful catapult could impart) and then uses its motor to climb to 1000 m, it has a lot of gravitational potential to exploit in gliding. With a 25:1 glide ratio, descending from 1000 m yields 25 km range without any power. Any solar or motor power can extend that dramatically – even a few kW can hold altitude or climb in thermals. Thus, trips on the order of 50–100 km seem very attainable on one charge. Longer trips, 200–300 km, might require some sunlight assistance or a bigger battery, but are still within what experimental electric planes have done. For example, the Sunseeker II (an earlier single-seat solar plane) once crossed the United States in stages, flying hundreds of kilometers on solar+battery each day . Our craft would benefit from not having to self-takeoff, saving a lot of energy.
In terms of safety comparisons, the target is to match or beat general aviation accident rates. Traditional small aircraft have fatal accident rates around 1-2 per 100,000 hours, whereas commercial airliners are far safer (~0.1 per 100,000 hours). Our system could potentially approach airline-level safety because of its simplicity (no complex engine or fuel, fewer parts to fail) and automation (removing pilot error). The presence of a ballistic parachute as a backup in every glider means even many of the worst-case failures won’t be fatal. This is a notable improvement over most GA planes which rely on pilot skill in emergencies. Additionally, launches and landings are in controlled, known locations – reducing unpredictability. We would design the system such that no single point of failurelikely leads to catastrophe: e.g., if the launch fails, the glider stays on ground; if the motor fails, it glides; if the computer fails, the backup takes over; if all else fails, the parachute deploys. By stacking these safety nets, the probability of a truly disastrous outcome becomes extremely low.
A potential drawback to consider is weather sensitivity. Lightweight gliders are more affected by strong winds and storms than heavy airliners. Operations might need to pause in high winds, thunderstorms, or icing conditions. This is similar to how helicopters or small planes sometimes can’t fly in extreme weather. We could mitigate this with scheduling (flights during favorable weather windows) and design (some weatherproofing, de-icing systems if needed for higher altitudes, etc.). The autonomous system would of course avoid flying into known storm cells and could delay or reroute flights as needed for safety. Over time, if we wanted all-weather capability, slightly heavier and more powerful versions of the craft could be developed (at the cost of some efficiency).
From a deployment cost standpoint, building electromagnetic launchers at scale is a significant infrastructure project. However, they may be comparable to building a new rail line or roadway interchange in cost, and possibly cheaper per mile of travel enabled. Each launcher site might cost tens of millions of dollars (considering EMALS on carriers cost ~$50–$100M each in R&D, though a simplified ground version could be less). The gliders themselves would likely be much cheaper than conventional aircraft – perhaps on the order of a family car or small airplane ($50k–$200k) once mass-produced, since they are mostly composite shells, a motor, and off-the-shelf electronics. Maintenance facilities and staff training will also factor in, but given the electric simplicity, maintenance could be streamlined with modular swaps (battery packs, motor units).
The legal and social acceptance will take time, but if positioned as a eco-friendly, high-tech form of transport that is as safe as an airliner, people may adopt it similar to how elevators and autonomous trains are now normal. Public demonstrations of the system working (perhaps televised launches showing how smooth and easy it is) will help. Also, early adopters might be tech enthusiasts and commuters in congested areas desperate for faster transport. If one can travel from one city to the next in a quarter of the time it takes by car or train, without emissions, and at a reasonable cost, the value proposition is strong.
In conclusion, the air-launch glider transit system marries ancient ingenuity with modern innovation. By catapulting solar-powered drones into the sky, we eliminate the runway and fuel requirements that have long constrained aviation. We harness the sun, electricity, and smart automation to let people fly between cities as naturally as taking a bus. Technically feasible with present or near-future technology, and with safety as the foremost design principle, this concept could transform short-range air travel. It offers a compelling alternative to both ground transport and conventional aircraft, one that is green, fast, and potentially very safe. Further detailed engineering and trials will iron out the remaining questions, but the pieces are in place for a 21st-century transportation network that fulfills the dream of flight – launched from the ground, open to all, and sustainable in the skies.
References and Technological Precedents
• EMALS (Electromagnetic Aircraft Launch System) – demonstrated smoother acceleration and ability to launch lighter craft than steam catapults .
• Wright Brothers’ catapult (1904) – proved ground launch could assist takeoff, using a 6 m tower and 600 kg weight to launch the Flyer .
• Ancient catapults – Greek/Roman catapults could hurl ~250 kg, conceptually enough to toss a glider (though inefficient in practice) .
• Winch-launched gliders – common in glider clubs; a modern winch can launch a sailplane to ~400 m altitude in seconds, conceptually similar to our system.
• Solar Impulse 2 – solar-powered airplane flew around the world (40,000 km) with no fuel , demonstrating endurance of solar-electric flight.
• Sunseeker Duo – two-seat solar plane that can cruise on solar power alone , empty weight 280 kg, 22 m wingspan – shows what lightweight solar craft can do.
• Wisk Cora autonomous eVTOL – fully autonomous air taxi prototype (no pilot onboard, no remote pilot) flying since 2017 ; equipped with an emergency parachute .
• Whole-aircraft parachutes – BRS and Cirrus CAPS have collectively saved hundreds of lives in small aircraft emergencies .
• FAA Part 103 Ultralight – legal framework allowing single-seat lightweight aircraft to be flown with no license , suggesting a path for license-free operation if weight/speed limits are met.
• Boeing 787 composites – first airliner ~50% by weight carbon-fiber, yielding ~20% weight reduction and higher strength , validating the use of composites for primary structure.
“Working with pharaohverse is becoming a game-changer for business. Our innovative solutions and collaborative approach have truly set us apart in the market.”
"Victor Claar II"
Create Your Own Website With Webador